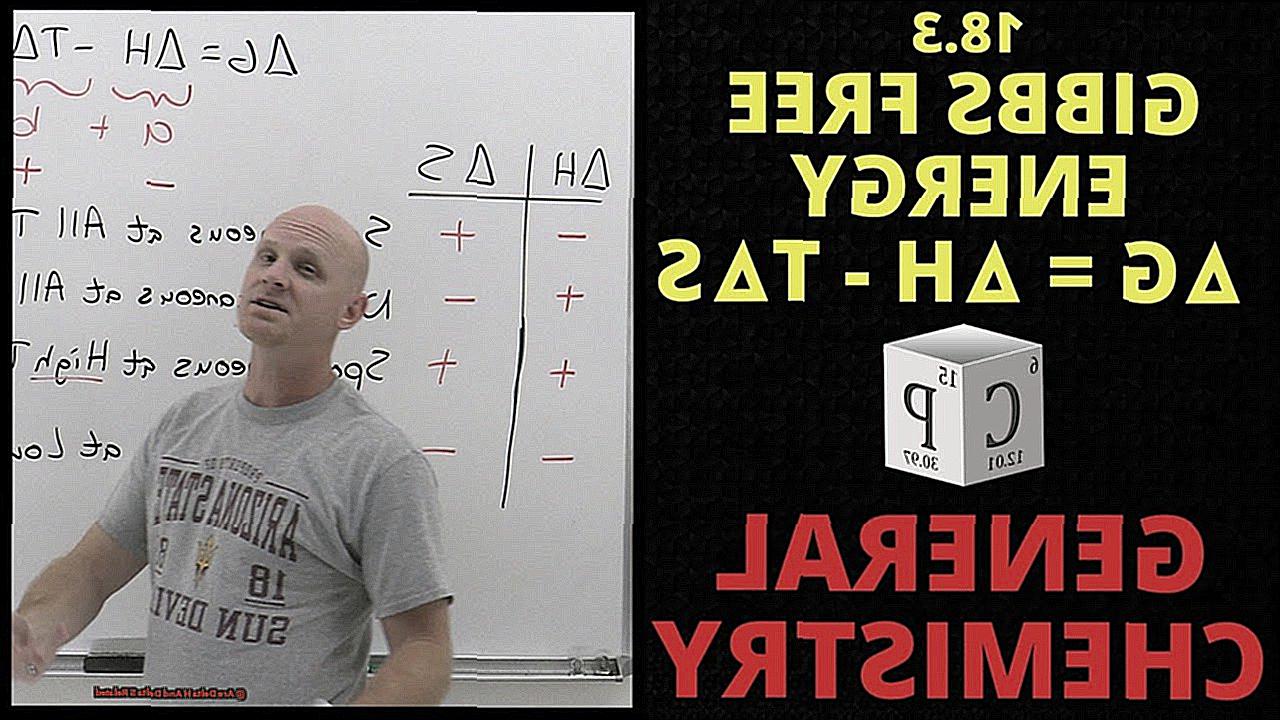
Ever wondered if Delta H and Delta S are BFFs or mortal enemies? Well, get ready to unravel the mystery. We all know chemistry can be mind-boggling, but understanding the relationship between these two thermodynamic rockstars will give you a whole new perspective.
Delta H, also known as enthalpy change, is like the heat transfer MVP in chemical reactions. It’s all about how much heat gets thrown around when atoms and molecules decide to party together. On the other hand, Delta S, or entropy change, measures how wild things get in terms of disorder and randomness. Think of it as the chaos factor.
But here’s the juicy part: Delta H and Delta S are actually connected. They’re like two sides of the same coin, working together to shape our chemical universe. In this blog post, we’ll dive deep into their fascinating dance and uncover how they influence each other.
So buckle up, my curious friends. We’re about to embark on an epic adventure into the captivating world of thermodynamics. Get ready for mind-blowing insights that will leave you saying “Whoa.” So grab your lab coat and safety goggles because it’s time to explore the incredible bond between Delta H and Delta S. Let’s go.
Contents
- 1 Are Delta H and Delta S related
- 2 Definition of Delta H and Delta S
- 3 The Relationship between Delta H and Delta S: The Gibbs Free Energy Equation
- 4 Spontaneity of Reactions: Negative Delta G
- 5 Exothermic Reactions: Negative Delta H and Positive Delta S
- 6 Endothermic Reactions: Positive Delta H and Negative Delta S
- 7 Temperature’s Role in the Relationship between Delta H and Delta S
- 8 Factors Influencing the Magnitude of Delta H and Delta S
- 9 Examples of Reactions with Different Relationships between Delta H and Delta S
- 10 Conclusion
The Relationship Between Delta H and Delta S: Exploring Spontaneity and the Gibbs Free Energy Equation
Have you ever wondered about the relationship between Delta H (enthalpy change) and Delta S (entropy change)? These two thermodynamic parameters play a crucial role in understanding the energy changes and randomness in chemical reactions and physical processes. In this blog post, we will explore their connection, focusing on spontaneity and the Gibbs free energy equation.
Spontaneity: A Key Factor in Chemical Reactions
Before we dive into the relationship between Delta H and Delta S, let’s understand the concept of spontaneity in chemical reactions. A spontaneous reaction is one that occurs without any external influence, meaning it can proceed on its own. In general, spontaneous reactions are characterized by a decrease in enthalpy (Delta H) and an increase in entropy (Delta S).
Enthalpy Change (Delta H): Heat Energy Exchange
Enthalpy change, represented by Delta H, measures the heat energy absorbed or released during a reaction or process. A negative Delta H value indicates that heat is released into the surroundings (exothermic reaction), while a positive Delta H value signifies heat absorption from the surroundings (endothermic reaction). These changes in enthalpy provide information about the energy flow in a reaction.
Entropy Change (Delta S): Disorder or Randomness
Entropy change, symbolized by Delta S, quantifies the degree of disorder or randomness in a system. A higher entropy value reflects greater disorder, while a lower entropy value indicates more order or organization. Reactions leading to an increase in the number of particles or gas molecules typically have a positive Delta S value, indicating an increase in entropy.
The Gibbs Free Energy Equation: Connecting Delta H and Delta S
To understand the relationship between Delta H and Delta S more comprehensively, we turn to the Gibbs free energy equation:
Delta G = Delta H – TDelta S
In this equation, Delta G represents the change in Gibbs free energy, Delta H represents the change in enthalpy, Delta S represents the change in entropy, and T represents the temperature in Kelvin. The sign of Delta G determines the spontaneity of a reaction.
Let’s explore the different scenarios based on the signs of Delta H and Delta S:
Negative Delta H and Positive Delta S: Exothermic and Disorder Increase
When Delta H is negative (exothermic) and Delta S is positive (increase in disorder), the reaction tends to be spontaneous. The negative Delta H contributes to a negative Delta G, indicating spontaneity. Moreover, the positive Delta S further drives the reaction towards spontaneity by increasing disorder.
Definition of Delta H and Delta S
These two parameters, enthalpy change and entropy change, together hold the key to understanding the spontaneity of chemical reactions. So let’s dive right in and unravel the intricacies of this energetic dance.
The Marvelous Duo: Delta H and Delta S:
Delta H, the change in enthalpy, represents the heat absorbed or released during a chemical reaction at constant pressure. It embodies the energy exchange between a system and its surroundings. On the other hand, Delta S signifies the change in entropy, which measures the disorder or randomness of a system. Think of Delta S as a measure of how “spacious” or “cramped” the energy of a system is.
The Gibbs-Helmholtz Equation:
To understand how Delta H and Delta S interact, we turn to the Gibbs-Helmholtz equation. This equation combines these two factors along with temperature (T) to determine the spontaneity of a reaction. It goes like this:
Delta G = Delta H – T * Delta S
Here, Delta G represents the Gibbs free energy, which reveals the maximum amount of work obtainable from a system at constant temperature and pressure.
The Dance of Spontaneity:
When Delta G is negative, the reaction is spontaneous, like a dance that flows effortlessly. In this case, if Delta H is negative (an exothermic reaction), an increase in entropy (Delta S > 0) will favor the spontaneity of the reaction. This means that as disorder increases, heat is released, and the reaction proceeds without external intervention.
Conversely, if Delta H is positive (an endothermic reaction), a decrease in entropy (Delta S < 0) will favor spontaneity. This suggests that as order increases, heat is absorbed, and the reaction proceeds spontaneously. However, when Delta G is positive, the dance falters, and the reaction becomes non-spontaneous. In these cases, an increase in enthalpy (Delta H > 0) or a decrease in entropy (Delta S < 0) contributes to the non-spontaneity of the reaction. The system resists change, requiring external influence to initiate the reaction.
Conclusion:
In this captivating dance of thermodynamics, Delta H and Delta S intertwine to determine whether a reaction is spontaneous or not. While Delta H represents the heat energy exchange, Delta S quantifies the degree of disorder or randomness. Together, they provide us with valuable insights into the thermodynamic behavior of a system.
The Relationship between Delta H and Delta S: The Gibbs Free Energy Equation
In the fascinating world of thermodynamics, the relationship between Delta H (change in enthalpy) and Delta S (change in entropy) takes center stage. Together, they perform a dance that determines the spontaneity and feasibility of chemical reactions. Let’s dive into the captivating interplay between these two factors and how they are combined in the Gibbs free energy equation.
Enthalpy: The Heat of the Dance
Enthalpy (Delta H) represents the heat absorbed or released during a chemical reaction. It measures the total energy content of a system. A negative Delta H signifies an exothermic reaction, where energy is released to the surroundings like a passionate burst of heat. Conversely, a positive Delta H indicates an endothermic reaction, where energy is absorbed from the surroundings, resulting in a cooler performance.
Entropy: The Chaotic Moves
Entropy (Delta S) is a measure of the disorder or randomness within a system. It quantifies how energy is distributed among various arrangements of particles. A positive Delta S indicates an increase in disorder, like a wild dance party where everyone moves freely. On the other hand, a negative Delta S represents a decrease in disorder, resembling a synchronized dance routine with limited movement options.
The Gibbs Free Energy Equation: Putting it All Together
The Gibbs free energy equation combines Delta H, Delta S, and temperature (T) to determine whether a reaction will spontaneously occur or need external intervention. It takes the form:
Delta G = Delta H – T * Delta S
Spontaneity: The Dance’s Tempo
The sign of Delta G determines whether a reaction is spontaneous or non-spontaneous. If Delta G is negative, the reaction is spontaneous and proceeds effortlessly like a well-choreographed dance routine. In this case, the reaction is exergonic, meaning energy is released. The magnitude of Delta G indicates the extent to which the reaction will proceed, with a larger negative value indicating a more favorable dance.
If Delta G is positive, the reaction is non-spontaneous and resists change. It requires the input of energy for the dance to begin. These reactions are endergonic, meaning energy is required for them to occur. The magnitude of Delta G provides information about the feasibility of the reaction, with a larger positive value indicating a less favorable dance.
At equilibrium, where Delta G is zero, the dance reaches a state of balance. The forward and reverse reactions occur at equal rates, symbolizing a harmonious coexistence of enthalpy and entropy changes.
Spontaneity of Reactions: Negative Delta G
In this article, we will explore how the Gibbs free energy change (ΔG) determines the spontaneity of a reaction when ΔH and ΔS have the same sign. So grab your dancing shoes and let’s dive in.
The Dance Partners: ΔH and ΔS
The Rhythm of Spontaneity
When ΔH and ΔS have opposite signs, determining spontaneity is straightforward. But what happens when they have the same sign? This is where the concept of Gibbs free energy change (ΔG) comes into play.
The Equation: ΔG = ΔH – TΔS
The equation for ΔG combines ΔH, ΔS, and temperature (T) to determine spontaneity. Let’s break it down:
Both ΔH and ΔS are positive:
- If TΔS > ΔH, the reaction is spontaneous.
- Why? The increase in disorder compensates for the absorption of heat energy, resulting in a negative ΔG.
Both ΔH and ΔS are negative:
- If TΔS < ΔH, the reaction is spontaneous.
- Why? The release of heat energy outweighs the decrease in disorder, leading to a negative ΔG.
Visualizing Spontaneity:
To help you visualize these scenarios, here’s a table summarizing their outcomes:
| ΔH | ΔS | Spontaneity |
|————|————|————-|
| Positive | Positive | Depends on the value of TΔS and ΔH |
| Negative | Negative | Depends on the value of TΔS and ΔH |
Exothermic Reactions: Negative Delta H and Positive Delta S
Today, we’re going to unravel the captivating relationship between enthalpy (ΔH) and entropy (ΔS) in exothermic reactions. Get ready to join me on the dance floor as we explore why some exothermic reactions have a negative ΔH value and a positive ΔS value. Let’s dive right in.
The Heat of the Moment:
Exothermic reactions are like firecrackers – they burst with energy. In these reactions, the reactants have higher energy levels than the products, resulting in a decrease in enthalpy (ΔH). This decrease is represented by a negative ΔH value, reflecting the heat released during the reaction. But wait, there’s more to this dance.
The Cha-Cha of Entropy:
Now, let’s sprinkle some spice into our routine by introducing entropy (ΔS). Picture entropy as the wild dancer, always seeking chaos and disorder. Positive ΔS values indicate an increase in the randomness and disorder of a system. But how does this fit into the exothermic reaction scene?
Finding Harmony in Dissolution:
One example of an exothermic reaction with a negative ΔH and positive ΔS is the dissolution of salts in water. As the salt dissolves, its ions separate and are surrounded by water molecules. This process increases the randomness and disorder (entropy) of the system, leading to a positive ΔS value. Meanwhile, energy is released as new interactions form between solute particles and water molecules, contributing to the negative ΔH value.
Sparks Fly in Combustion:
Another example that showcases this intriguing dance is combustion reactions. Imagine a fiery tango between fuel and oxygen as they create carbon dioxide and water while releasing heat. The breaking of bonds in the fuel molecules requires energy, but fear not, for new bonds are formed in the products, releasing even more energy. This release of energy leads to a negative ΔH value. Additionally, combustion reactions involve an increase in the randomness and disorder of the system, resulting in a positive ΔS value.
The Grand Finale:
In summary, exothermic reactions with negative ΔH values and positive ΔS values demonstrate the intricate relationship between enthalpy and entropy. While exothermic reactions generally have a negative ΔH value due to the release of energy, their ΔS value can vary depending on the specific process involved. Remember, it’s not just about the heat – entropy has its own moves on the dance floor.
Endothermic Reactions: Positive Delta H and Negative Delta S
In the world of chemistry, there is a delicate dance between enthalpy (Delta H) and entropy (Delta S). While exothermic reactions with negative Delta H and positive Delta S values are more commonly discussed, endothermic reactions with positive Delta H and negative Delta S values can also occur spontaneously. In this article, we will explore how these seemingly contradictory reactions can still happen and the factors that influence their spontaneity.
Understanding Endothermic Reactions:
Endothermic reactions absorb heat from the surroundings, resulting in an increase in the enthalpy of the system. This means that the products of the reaction have higher energy content than the reactants. On the other hand, a negative Delta S value indicates a decrease in the entropy or disorder within the system. This could be due to reactant molecules coming together to form more ordered products or a decrease in the number of moles of gas involved in the reaction.
The Gibbs Free Energy Equation:
To understand how endothermic reactions with positive Delta H and negative Delta S values can still occur spontaneously, we can turn to the Gibbs free energy equation:
Delta G = Delta H – T * Delta S
In this equation, Delta G represents the change in free energy of the system, T denotes temperature, and Delta H and Delta S represent enthalpy and entropy changes, respectively. According to this equation, when Delta G is negative (indicating a spontaneous reaction), a positive Delta H value can be offset by a sufficiently negative T * Delta S value.
Factors Influencing Spontaneity:
Several factors come into play when determining whether an endothermic reaction with positive Delta H and negative Delta S will occur spontaneously:
- Magnitude of Delta H and Delta S: The magnitude of these values is crucial. If the positive Delta H value is too large or the negative Delta S value is too small, the reaction may not be spontaneous, even with a negative Delta G.
- Temperature: The temperature at which the reaction occurs also plays a significant role. As temperature increases, the T * Delta S term becomes more significant, potentially offsetting the positive Delta H value and making the reaction spontaneous.
- Other Factors: It’s essential to consider other factors such as pressure, concentration, and catalysts that may influence the spontaneity of the reaction. These factors can affect the overall energy balance and entropy change, ultimately impacting whether the reaction occurs spontaneously or not.
Temperature’s Role in the Relationship between Delta H and Delta S
In the captivating world of chemistry, temperature plays a crucial role in determining the spontaneity of a reaction. The relationship between Delta H (enthalpy) and Delta S (entropy) is influenced by temperature, leading to fascinating insights into the thermodynamics of chemical reactions. Let’s explore the temperature’s role in this delicate dance between enthalpy and entropy.
Temperature’s Impact on Entropy:
At higher temperatures, molecules possess more kinetic energy, resulting in increased collisions and greater disorder within the system. This leads to an increase in entropy (Delta S). Conversely, at lower temperatures, molecules have less kinetic energy, resulting in fewer collisions and less disorder, leading to a decrease in entropy.
The Gibbs Free Energy Equation:
The relationship between Delta H, Delta S, and temperature can be described by the Gibbs free energy equation: Delta G = Delta H – T * Delta S. Here, Delta G represents the overall free energy change, T is the temperature in Kelvin, and Delta H and Delta S represent changes in enthalpy and entropy.
Dominance of Enthalpy at Low Temperatures:
At low temperatures, the T * Delta S term becomes relatively small compared to Delta H. In this case, the enthalpy change dominates and determines the spontaneity of the reaction. A positive Delta H (endothermic) can make the overall free energy change positive (non-spontaneous), while a negative Delta H (exothermic) can make it negative (spontaneous).
Entropy’s Influence at High Temperatures:
As temperature increases, the T * Delta S term becomes larger and can have a significant impact on the overall value of Delta G. At high temperatures, even if Delta H is positive (endothermic), if the increase in entropy (Delta S) is large enough, it can make the overall free energy change negative (spontaneous).
Non-Linear Relationship:
It is important to note that the relationship between Delta H and Delta S is not linear. The impact of temperature on the overall free energy change depends on the specific values of Delta H and Delta S for a given reaction. The magnitude of Delta H and Delta S determines whether temperature will enhance or diminish the effect of entropy on spontaneity.
Factors Influencing the Magnitude of Delta H and Delta S
Chemistry, the captivating world of molecules and reactions, is like a symphony where temperature conducts the delicate dance between enthalpy (Delta H) and entropy (Delta S). The interplay between these thermodynamic parameters determines the spontaneity and directionality of chemical reactions. In this blog post, we will explore the factors that influence the magnitude of Delta H and Delta S, shedding light on the fascinating complexities of heat, disorder, and energy changes.
Nature of Reactants and Products:
The types of molecules involved in a reaction greatly impact the magnitude of Delta H and Delta S. Reactions involving strong bonds tend to exhibit larger enthalpy changes compared to reactions with weaker bonds. Additionally, reactions leading to an increase in the number of gas molecules result in larger entropy changes because gases are inherently more disordered than liquids or solids.
Temperature:
Temperature plays a significant role in determining the magnitude of Delta H and Delta S. As temperature rises, molecules gain kinetic energy, leading to more energetic collisions and higher reaction rates. This can result in larger enthalpy changes. Moreover, at higher temperatures, molecules have increased thermal energy to explore different positions and orientations, leading to greater disorder and larger entropy changes.
Pressure:
Changes in pressure influence the magnitude of Delta H and Delta S, particularly in gas-phase reactions. An increase in pressure favors reactions that decrease the number of gas molecules, resulting in smaller entropy changes. Conversely, a decrease in pressure favors reactions that increase the number of gas molecules, leading to larger entropy changes.
Concentration:
The concentration of reactants and products affects the magnitude of Delta H but not Delta S directly. Higher concentrations generally lead to larger enthalpy changes due to increased frequency of collisions between molecules. However, entropy is related to the number of microstates available to the system, rather than the concentration of molecules.
Catalysts:
The presence of a catalyst can significantly influence the magnitude of Delta H and Delta S by providing an alternative reaction pathway with lower activation energy. Catalysts do not affect the enthalpy change (Delta H) but can increase the rate at which equilibrium is achieved. This indirect impact on equilibrium position can influence the magnitude of entropy changes if it shifts towards a greater or lesser number of gas molecules.
Phase Changes:
Phase changes, such as melting, vaporization, and condensation, involve significant energy and entropy changes. These processes exhibit large enthalpy changes (Delta H) due to the breaking or formation of intermolecular forces. Additionally, phase changes often result in large entropy changes (Delta S) as the system’s randomness and disorder increase or decrease.
Examples of Reactions with Different Relationships between Delta H and Delta S
Today, we’re diving into the captivating world of Delta H and Delta S, the dynamic duo that determines the fate of chemical reactions. Prepare to be amazed as we explore examples of reactions with different relationships between these thermodynamic parameters. Let’s get started.
Exothermic Reactions with Positive Delta S:
Our first example is the fiery combustion of methane (CH4) to form carbon dioxide (CO2) and water vapor (H2O). This exothermic reaction not only releases a blazing amount of heat but also leads to an increase in entropy. How? Well, during this reaction, one molecule of methane transforms into multiple molecules of carbon dioxide and water vapor, adding a touch of disorder to the equation. So, we have a negative Delta H (heat release) paired with a positive Delta S (increase in entropy).
Endothermic Reactions with Negative Delta S:
Now, let’s cool things down a bit. Picture the decomposition of calcium carbonate (CaCO3) into calcium oxide (CaO) and carbon dioxide gas (CO2). This endothermic reaction absorbs heat from its surroundings while simultaneously decreasing entropy. As the reactant decomposes into two products, order triumphs over chaos. Thus, we have a positive Delta H (heat absorption) alongside a negative Delta S (decrease in entropy).
Reactions with Negative Delta H and Delta S:
Get ready for some electrifying chemistry. The formation of sodium chloride (NaCl), that savory seasoning we all love, showcases a spontaneous reaction with negative values for both Delta H and Delta S. As sodium (Na) donates an electron to chlorine (Cl2), heat is released, warming our taste buds. Simultaneously, the formation of an ordered crystalline structure results in a decrease in entropy. But fear not. The heat’s release compensates for the decrease in disorder, making this reaction spontaneous.
Reactions with Positive Delta H and Delta S:
Let’s heat things up, quite literally. Imagine the mesmerizing transformation of liquid water (H2O) into gaseous water vapor (H2O(g)). This vaporization process requires an input of heat energy, making it endothermic with a positive Delta H.
But here’s the twist – as water molecules break free from their liquid prison, they indulge in an exuberant dance of disorderliness, increasing entropy.
So, we have a positive Delta H (heat absorption) paired with a positive Delta S (increase in entropy) that ultimately makes this reaction spontaneous at higher temperatures.
Conclusion
In conclusion, it is clear that Delta H and Delta S are indeed related.
The change in enthalpy (Delta H) and the change in entropy (Delta S) both play crucial roles in determining the spontaneity of a chemical reaction. When Delta H is positive and Delta S is negative, the reaction tends to be non-spontaneous.
This relationship between Delta H and Delta S can be explained by the second law of thermodynamics, which states that for a process to occur spontaneously, the total entropy of the system and its surroundings must increase.